Technology sometimes unfolds at a slow, measured pace and sometimes at lightning speed. Right now, we are witnessing what is arguably one of the fastest moving fields in biomedical science: a form of genome editing aptly known as CRISPR.
CRISPR allows researchers to make very precise—some would say crisp—changes to the genomes of human cells and those of other organisms. You might think of it as a kind of guided missile. Its precision is opening the doors to a wide variety of research and, hopefully, medical applications. Indeed, the possibilities seem to be bound only by scientists’ imaginations.
“For a long time, we have been accumulating new knowledge about which gene mutation causes which disease. But until very recently, we haven’t had the ability to go in and correct those mutations,” explains Feng Zhang, PhD, a core member of the Broad Institute of Harvard and MIT, and one of the method’s pioneers. “CRISPR is one of the tools that is starting to allow us to directly go in and do surgery on the genome and replace the mutations.”
CRISPR stands for Clustered Regularly Interspaced Short Palindromic Repeats. While this name is a bit verbose, it points to the technology’s origins: a set of genetic sequences first discovered in bacteria.
In the late 1980s, a group of Japanese researchers stumbled across an unusually repetitive sequence at the end of a gene in Escherichia coli bacteria. Little was thought of it at the time, but fast-forward about a decade. Through widespread sequencing of microbial genomes, it became clear that many microbes share these mysterious repeats.
For a while, the general wisdom was that these CRISPR sequences were of no functional significance—basically, junk DNA. But scientists eventually learned that they formed a kind of genomic memory. Just as our immune system remembers the pathogens it’s fought, CRISPR sequences give bacteria a physical record of the viruses that bacteria had come across. (Yep, viruses can plague bacteria, too.)
The powers of Cas9
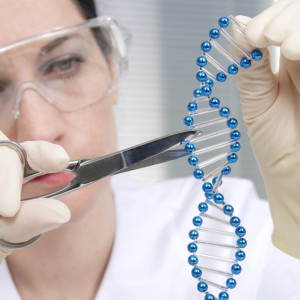
Like the scissors in this picture, the enzyme Cas9 cuts DNA. It can be programmed to find and bind to specific sites in the genome.
Zhang’s team proved that it worked in human and mouse cells. George Church, PhD, another CRISPR pioneer and a professor of genetics at Harvard Medical School, accomplished a similar feat.
“There are other technologies that allowed people to make genome changes, but they have been very technically challenging to use,” says Zhang, who is also the W. M. Keck Career Development Professor in Biomedical Engineering at MIT and an investigator at the McGovern Institute for Brain Research.
Therapeutic editing
In just the last couple of months, scientists have published a flurry of papers describing applications of CRISPR:
- Church and his colleagues described a method for harnessing CRISPR—known as “gene drives”—that can be leveraged across many species. For example, it could be used in mosquito populations to aid in malaria control.
- A team led by researchers at Temple University wielded CRISPR to remove the genetic code of HIV from infected cells. The work, using human cell lines, builds upon research published by a Japanese team last year, and points to a new approach to HIV treatment.
- A holy grail in biomedicine is to create induced pluripotent stem cells (iPS cells) from patients, repair the genetic defect and use these corrected cells to cure the disorder. Researchers from the University of California, San Francisco, reported some initial encouraging steps along this path for beta-thalassemia, a common blood disease that stems from mutations in the hemoglobin beta gene. Applying CRISPR to sickle cell anemia, George Q. Daley, MD, PhD, and colleagues at Boston Children’s Hospital are tackling the challenge of producing mature red blood cells from corrected iPS cells to prove that genome editing repairs their tendency to sickle.
- MIT scientists used CRISPR to directly engineer cancer-causing mutations in the livers of mice, providing a rapid way to create mouse models of cancer. As massive genome-wide hunts unearth vast numbers of cancer-associated mutations, having a method to quickly test them functionally will be critical.
- Last month, scientists at the University of Texas Southwestern Medical Center reported the use of CRISPR to correct the dystrophin gene and block the onset of Duchenne muscular dystrophy in a mouse model of the disease.
While these findings and others are turning heads, let us not forget that the CRISPR technology is young. Barely two years old, it is a veritable infant, and its clinical use is at least several years away. Nevertheless, researchers have high hopes for its future.
“Hopefully, we’ll be able to get the technology to a level where you can actually make a difference in a patient’s life,” said Zhang.
Nicole Davis, PhD, is a freelance writer and communications consultant specializing in biomedicine and biotechnology. She has worked in science communications for nearly a decade and earned her PhD in genetics at Harvard University.