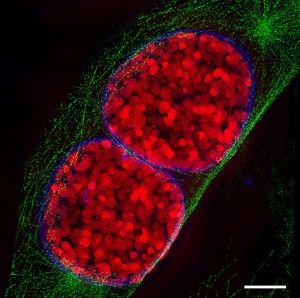
While a cell's chromosomes (red, in a dividing cell) may look like they sit in a tangled jumble, they are actually organized in precise 3D fashion, which in turn influences what happens when chromosomes break. (Lothar Schmermelleh/Wikimedia Commons)
We’ve known for decades that our chromosomes can break and reshuffle, especially in cancer cells. We also see this process, called translocation, in naïve B cells when they start to produce antibodies for the first time: the cell breaks, shuffles and recombines genes to decide which threat it will defend against.
But knowing these things happen doesn’t mean that we’ve understood the rules for how and why they happen. By combining two powerful methods of genomic mapping, a research team led by Frederick Alt, director of the Program in Cellular and Molecular (PCMM) Medicine at Children’s Hospital Boston and the Immune Disease Institute (IDI), has brought some of those rules into clearer focus. It turns out that the genome’s three-dimensional organization – where each of the genome’s thousands of genes lie spatially within the cell’s nucleus – holds great influence over where broken chromosome ends rejoin.
“While chromosomal breaks and translocations are fundamental to many cancers, historically we’ve had no approaches to systematically study how they are generated,” says Alt. “About five years ago, our group set out to generate a high-throughput approach to address this important problem in cancer biology.”
To accomplish this goal, the Alt lab developed high-throughput genome-wide translocation sequencing (HTGTS). This technique maps “hot spots” in the genome, places where chromosome breaks and translocations are more likely to occur. In early HTGTS studies, they found that broken chromosomes often rearrange within themselves, as opposed to sharing pieces across different chromosomes.
To probe these findings more deeply, Alt’s laboratory joined forces with Job Dekker – co-director of the Program in Systems Biology at the University of Massachusetts Medical School – to combine HTGTS with another method called Hi-C. Developed by Dekker’s group, Hi-C measures how all the sequences in the genome are arranged relative to one another in three dimensions.
What they found were several related but distinct principles that together highlight the relationship between proximity (how close two chromosomes or genes are physically), the genome’s 3D structure, and break frequency (the likelihood that a chromosome will break at a given gene sequence).
“Two sequences have to be broken and physically proximal to join,” Alt explains. “If two sequences are together in most cells and frequently broken, they will translocate in many cells. If two sequences are frequently together but one of them doesn’t break, or if they both break frequently but always lie on opposite sides of the nucleus, the chances that they will translocate are very low or zero.
“However, if both sequences break very frequently and are close together in only a subset of cells,” he continues, “they will very frequently translocate in that subset, contributing to recurrent translocations.” This last point, he notes, helps explain the recurrent translocations often seen in lymphomas.
This new understanding of the relative roles of proximity, organization and frequency in chromosomal translocations opens up new avenues for deciphering how the organization of cell’s nucleus affects the genomic disarray found in cancer and other diseases characterized by chromosome reshuffling. “We feel that our findings and the application of our approaches will provide a new lens through which to view the genomes of many different types of cancer,” Alt says.
[Ed. note: To learn more, check out an article posted by the IDI.]