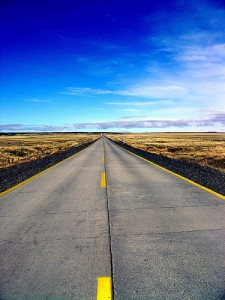
The different gene segments your immune system needs to build a diverse selection of antibodies are spread out across huge genomic distances. Frederick Alt may have found the genetic switch that helps manage all of the far flung pieces. (Jeremy Vandel/Flickr)
Imagine for a moment, that you are your immune system. On any given day, you’re faced with host of threats: a virus here, a bacterium there, a new fungus. And don’t forget those wayward cells lurking around the corner, the ones that might become a tumor.
Now, you have to respond to these challenges, but how you do it? Each looks different, meaning that you have to produce a new T or B cell (your two main tools) that can find, mark and guide the attack against each new threat.
Luckily, your T and B cells can turn to three sets of gene segments that, together, contain the genetic raw material for the variety you need. Called the variable (V), diversity (D) and joining (J) segments, they are constantly cut up, shuffled and rejoined by the genome to make new genes – a process called V(D)J recombination – for new receptors (on T cells) or antibodies (from B cells), giving your immune system the most diverse arsenal possible.
And that diversity is astounding. For one component of the average human antibody alone, called the heavy chain, 65 V, 27 D and six J segments are found in clusters spread out across a huge swath of chromosome 14. It’s estimated that with all of the possible combinations of V, D and J segments, the immune system has the ability to react to at least 100 million forms of attack.
But how do our cells manage this diversity while making sure that they can respond to each threat with pinpoint accuracy? Especially with gene segments spread out so far on the chromosome? It is a daunting task, and researchers have long sought the underlying principles and mechanisms by which the Vs, Ds and Js are reshuffled.
By studying mouse antibody genes, a team led by Frederick Alt of the Immune Disease Institute and the Program in Cellular and Molecular Medicine at Children’s may have found the answer: a set of regulatory genes nestled in a stretch of mouse chromosome 12 amid the clusters of V and D gene segments that he and his collaborators have dubbed InterGenic Control Region 1 (or IGCR1).
The Alt lab has amassed a progressive body of knowledge about the rules of antibody diversity and production for nearly 30 years. They’ve found, for instance, that V, D and J genes are reshuffled in a specific order (Ds join to Js, and then D+Js join to Vs); that only one enzyme is responsible for generating all of these cutting and joining events; and that the regulation occurs at the level of joining the V to the DJ segments (despite their genomic distance). Those discoveries led them to suspect that whatever controls antibody diversity lies between the clusters of V and D genes. Systematic studies led by Chunguang Guo and Hye-Suk Yoon in Alt’s lab, showed that IGCR1 fits the bill.
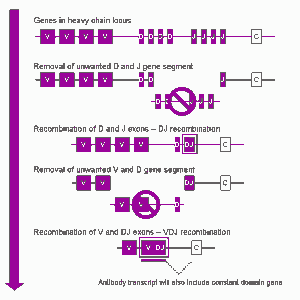
In this diagram, IGCR1 would stand as a barrier between the clusters of V and D gene segments, preventing premature recombination. (gustavocarra/Wikimedia Commons)
So how does IGCR1 work? Alt proposes a complex model in which it stands as a barrier between the V and D segments, stopping them from recombining before the time is right. Take that barrier away, and the immune system loses both diversity and specificity: as a group, the B cells as a group to produce a less diverse repertoire of antibodies; individually, they gain the capacity to produce more than one kind of antibody at a time (whereas mature B cells normally produce a single type of antibody against a single target).
The discovery of IGCR1’s role in maintaining and controlling antibody diversity answers some long-standing questions about how B cells control antibody production across a far-flung set of genes, giving the flexibility and specificity to protect us from much of what would harm us. It may do even more, providing a glimpse into the larger rules that govern how genes interact across large stretches of the genome as a whole, a concept the Alt lab is pursuing.