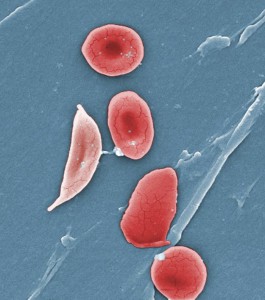
Flipping a single molecular switch could turn off the mutation that causes sickle cell diseae. Stuart Orkin has already done it in mice. (CDC PHIL)
What if we really could turn our bodies’ clocks back? In some cases, that could be a really good thing. Take sickle cell disease. A scourge of tens of thousands worldwide, it stems from a genetic defect in hemoglobin, the oxygen-carrying protein in red blood cells.
Normally, our bodies can produce two forms of hemoglobin: adult hemoglobin, the form susceptible to the sickle cell mutation; and fetal hemoglobin, which is largely produced during development and for a short time after birth. Our bodies finish making the switch from fetal to adult hemoglobin production by about four to six months old – the same time frame when children with the sickle cell mutation first start to show symptoms of the disease.
Fetal hemoglobin is not affected by the sickle cell mutation, and if produced at a sufficient level can compensate for the mutated adult form: Those rare individuals who have the sickle cell mutation but who naturally carry high levels of fetal hemoglobin in their bloodstream tend to have few or very mild symptoms.
“We know from clinical studies that the body needs to produce cells containing only 15 to 20 percent hemoglobin to reverse sickle cell disease,” says Stuart Orkin, associate chief of the division of hematology/oncology at Children’s Hospital Boston and chair of pediatric oncology at Dana-Farber. Orkin has studied sickle cell disease and related blood disorders like β-thalassemia for three decades.
It stands to reason that flipping that switch back and making red blood cells act young again might reverse the disease. The first clues about which switch to flip came in 2008, when genome-wide association studies of sickle cell disease patients highlighted the gene for a transcription factor called BCL11A. The following year, Orkin and his colleagues established BCL11A’s role as the fetal-to-adult switch, showing that by genetically knocking it out they could activate fetal hemoglobin and silence mutated adult hemoglobin in vitro in human red blood cell precursors.
Orkin and his team recently took the next step: By turning the gene off, they were able to correct sickle cell disease in two different mouse models. Eighty-five percent of the red blood cells in those mice carried fetal hemoglobin, and on average, 30 percent of the hemoglobin contained within those cells was of the fetal type – far more than necessary to reverse the disease. What’s more, the mice showed none of the clinical features of sickle cell, and their red blood cells looked normal: no sign of sickling.
“With these results we know now we have a target that, if we can develop ways to inactivate or silence it clinically, could be very beneficial to people with sickle cell,” Orkin says. “We also are screening the protein against libraries of chemicals in the hope of identifying compounds that interfere with BCL11A, and think it may also hold promise as a target for genetic therapies.”
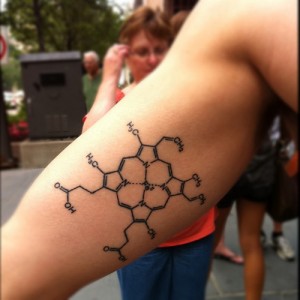
Both the sickle cell and β-thalassemia mutations disrupt the beta subunit of hemoglobin (above). (Unlisted Sightings/Flickr)
BCL11A may have roles to play in other blood disorders like β-thalassemia. It too arises from an inheritable hemoglobin defect, albeit a different one than sickle cell disease. And it manifests itself as a severe anemia that also arises in the first year of life – the same time frame as sickle cell and the fetal-to-adult hemoglobin switch.
Orkin and Vijay Sankaran, a clinical fellow in his lab, recently published a study of three families with unusual forms of β-thalassemia. Members of all three had the clinical features of β-thalassemia, but with uncommonly high levels of fetal hemoglobin. Sankaran analyzed the hemoglobin genes and their neighbors, finding that the three families were missing a stretch of DNA between the genes for two of adult hemoglobin’s component chains – in the region where, as it turns out, BCL11A happens to attach.
The loss of its binding site meant that the transcription factor couldn’t carry out its normal task of silencing fetal hemoglobin, explaining why these families carried so much more in their red cells, while highlighting the importance of this region of the genome for controlling hemoglobin production.
“Finding these families was a real boon,” says Sankaran. “It gave us the opportunity to show how matching genetic maps to clinical characteristics can tell us a lot about how we regulate hemoglobin, and how that relates to human diseases like thalassemia.”
[Ed. Note: Sankaran will speak about BCL11A, sickle cell anemia, and the thalassemias this Friday, Oct. 21, at Children’s 2nd Annual Rare Disease Symposium. Learn more from the Children's Hospital Boston Technology and Innovation Development Office.]