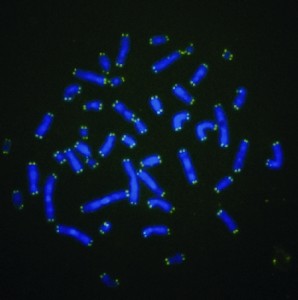
The green tips of these chromosomes are telomeres, whose length is a measure of cellular "aging" and determines how many times a cell can divide.
In a roomful of kids’ cancer specialists, like those listening to the keynote speech by George Daley, closing an international pediatric oncology meeting in Boston, the Myc gene is better known as a mutated weapon of mass destruction.
But this driver of cancer growth is also part of a four-gene cocktail that can reprogram an adult skin cell back into an embryonic-like stem cell that holds great therapeutic potential.
Daley’s team was among the first to report the creation of induced pluripotent stem cells (iPS cells) in human cells. “All it takes is a skin biopsy or a simple blood draw to begin to realize the full potential for any patient,” Daley said.
Bone marrow transplants from donors already save the lives of some kids with cancer. But Daley sees a future where genetic diseases can be cured by creating iPS cells from a child’s own diseased cells, rendering them disease-free through genetic intervention, then growing them into healthy tissue that won’t be rejected by the patient’s immune system. “The goal is to create customized patient-specific stem cells,” Daley said.
The road to transplantation therapy begins with understanding what diseased cells can be transformed into iPS cells, how transformation affects the cells, and how the genetic defects can be fixed. “For now, iPS cells are tools for research into disease mechanisms and tools for drug discovery,” Daley said.
Daley reviewed his group’s work with several bone marrow failure syndromes in children that predispose them to cancer. The research has resulted in unexpected findings and prospects for regenerative medicine therapy.
In one effort, a team led by Suneet Agarwal, an attending physician in hematology at Children’s Hospital Boston, observed reprogrammed cells that seemed to cure themselves. They reprogrammed cells from a 3-year-old girl with a mitochrondrial disease known as Pearson marrow pancreas syndrome. In a lab dish, her iPS cells slowly segregated and, on their own, purged the mutant mitochrondrial DNA. From the purged cells, Agarwal’s team generated disease-free blood stem cells without any detectable mutant mitochondrial DNA. This biological phenomenon might explain how some patients outgrow their need for blood transfusions.
A second bone marrow failure syndrome, dyskeratosis congenita, features mutations in the gene for an enzyme that maintains telomeres, extensions of chromosomes that normally diminish with each cell division. The iPS cell reprogramming process works in part by activating this fountain-of-youth enzyme — so Daley’s team wondered if the disease defect would make cells resistant to reprogramming.
Surprisingly, it didn’t. In fact, Daley reported, Agarwal found that the pluripotent state actually reversed the premature senescence of the diseased skin cells. The iPS cells steadily regrew their telomeres. The pluripotent state overrode the limiting genetic lesions, suggesting potential future therapy to replicate the enzymatic activity.
In contrast, mutated cells from yet another fatal genetic blood disease, Fanconi anemia, resisted reprogramming efforts. “After a year of trying, we became enormously frustrated,” Daley said. “We were even more frustrated when we were scooped by competitors who had run into similar resistance until they first performed a gene correction on the cell.”
Daley and his colleagues at Dana-Farber/Children’s Hospital Cancer Care will get another shot at Fanconi anemia. Last month, the National Institutes of Health gave them a three-year, $3.9 million grant to develop a therapy to treat Fanconi anemia.
“The grant is aimed at defining precisely what mechanism is lacking in Fanconi cells that is required for efficient reprogramming,” said Daley via email. “We believe the DNA repair defects of Fanconi cells are a problem—because the reprogramming technique may stress the DNA repair response; thus, being defective in Fanconi anemia cells makes these cell relatively resistant to reprogramming.”
To realize the vision of future cures for bone-marrow disorders and other genetic diseases, many more challenges need to be overcome: the genetic and epigenetic instability of cells grown in lab dishes, inefficient genetic repair, directing differentiation of the pluripotent cells and getting the repaired cells and tissues to engraft in patients. Not to mention that the most common technique for cellular reprogramming, using viruses to deliver the reprogramming genes, carries the risk of causing oncogenic mutations in the reprogrammed cells, said Daley. (He cited a recent advance using RNA, instead of DNA, to reprogram skin cells into iPS cells.)
For more about Fanconi anemia and the grant work, read this related post.