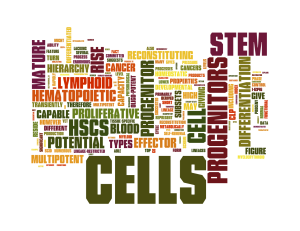
The demand for hematopoietic stem cell transplants is rising. But how can we get more cells? (Text from Bryder D, Rossi DJ and Weissman IL. Am J Pathol 2006; 169(2): 338–346.)
There are many HSCs in the bone marrow, but getting them out in sufficient numbers is laborious—and for the donor, can be a painful process. Small numbers of HSCs circulate within the blood stream, but not nearly enough. And while umbilical cord blood from newborn babies may present a relatively rare but promising source for HSCs, a single cord generally contains fewer cells than are necessary.
And here’s the rub: The demand for HSCs is only going to increase. Once a last resort treatment for aggressive blood cancers, HSCTs are being used for a growing list of conditions, including some solid tumor cancers, non-malignant blood disorders and even a number of metabolic disorders.
So how do we get more blood stem cells? Several laboratories at Boston Children’s Hospital and Dana-Farber/Boston Children’s Cancer and Blood Disorders Center are approaching that question from different directions. But all are converging on the same end result: making more HSCs available for patients needing HSCTs.
One of these progenitors is not like the others
To grow more stem cells, you have to begin with the right starting material, which requires an understanding of the nature of the stem and progenitor cells that give rise to all of the blood’s components. The pathways from embryonic stem cell to red and white blood cells were mapped years ago, but new work by Stuart Orkin, MD, of Dana-Farber/Boston Children’s, is revealing minute differences within the hematopoietic hierarchy—the different stages of blood development—at unprecedented detail.
With help from postdoctoral fellow Guoji Guo, PhD, Orkin is mapping the surface markers cells display over the course of development. But instead of looking at the surfaces of populations of cells, Guo and Orkin are looking within, using a multiplexed polymerase chain reaction assay to measure the expression of the genes encoding 280 common surface markers.
All at once. In individual cells.
As the pair showed in Cell Stem Cell, the assay highlighted a striking amount of heterogeneity at each level of the hematopoietic hierarchy. They’re also finding new branches in the conventionally accepted pathways.
“We’re seeing new hierarchies that differ from the classic one,” Guo says. “For instance, we see the cells that give rise to red blood cell and platelet progenitors split off much earlier than was previously thought. Such discoveries could be done now, with computational analysis of single cell data.”
Flipping the right switches
Derrick Rossi, PhD, of the Boston Children’s Program in Cellular and Molecular Medicine, also wants to know how different blood stem and progenitor cells function, but from a different viewpoint. With an international group of researchers called the Immunological Genome Project Consortium (ImmGen), Rossi studies the genes that run these cells’ internal machinery.
In a recent paper in Stem Cell Reports, Rossi and the ImmGen group reported that they could tell different blood stem and progenitor populations apart based on their unique transcriptomes—the pattern of genes turned on and off. In the process, they identified 51 transcription factors (genetic switches that regulate gene activity) that are most highly expressed in HSCs.
When they activated one of those factors, called Hlf, in cells further down the hematopoietic hierarchy, the researchers found that those cells started to display surface markers associated with HSCs. The Hlf-augmented cells also behaved like HSCs, renewing themselves and giving rise to many different blood and immune cell lineages.
“Transcription factors define what a cell is,” Rossi says. “Our question was, is there a pattern that defines HSCs versus everything else? We found the transcription factor genes are mostly uncharacterized, because HSCs are so hard to get. But given our functional results with Hlf, it looks like we’ve found something pretty cool.”
Putting iPSCs to work
Clockwise from top left: George Q. Daley, MD, PhD; Stuart Orkin, MD; Derrick Rossi, PhD; Leonard Zon, MD
But rather than reprogramming a skin cell or B lymphocyte, their strategy for re-specification starts with a blood progenitor like common myeloid progenitors—cells that are functionally “closer” to the desired end product (namely, HSCs) than skin or B cells.
“It’s like being at an archery range,” says Sergei Doulatov, PhD, a postdoctoral fellow in Daley’s lab in Boston Children’s Stem Cell Research Program. “If you move the target closer to you, you increase your chances of hitting a bull’s-eye.”
As they reported in Cell Stem Cell, the pair took induced pluripotent stem cells (iPSCs) that had been differentiated into myeloid progenitors. They then used a select set of transcription factors to turn the molecular clock back on these committed myeloid cells just a little, producing HSC-like cells that readily engrafted and differentiated when transplanted into mice.
The pair hopes that re-specification may help overcome technical barriers to generating blood disease-specific animal models from the thousands of iPSC cell lines now sitting in laboratory freezers around the world (until now, no one has been able to get iPSCs to differentiate into transplantable cells for in vivo modeling). And the technique also may hold future promise for producing cells suitable for transplant.
Finding their way home
Orkin, Rossi and Daley all want to make more HSCs. Leonard Zon, MD, wants to make better use of the cells we already get.
Six years ago, Zon’s laboratory (also in the hospital’s Stem Cell Research Program) announced that they’d found that a compound called prostaglandin E2 (PGE2) boosted blood stem cell numbers in zebrafish—a popular model organism with genetic features strikingly similar to our own. Subsequent work showed that the compound didn’t make blood stem cells grow more, but rather helped blood stem cells migrate more effectively to the bone marrow.
“We think PGE2 acts as a kind of priming mechanism,” Zon said. “It gets the cell set, so it will function better once it is introduced into the recipient’s body.”
Those preclinical findings fueled a recently completed Phase I clinical trial—the first-ever clinical trial of a drug identified in zebrafish—of umbilical cord blood transplants using cells treated with ProHema®, a form of PGE2 being developed by Fate Therapeutics.
The trial, published in Blood by a team led by Corey Cutler, MD, PhD, of Dana-Farber Cancer Institute, showed that ProHema was safe and helped the transplanted cells engraft and rebuild the patients’ blood more quickly.
Bringing it all back together
And now back to our original question: How can we get more blood stem cells? No single method being worked on here or at other laboratories worldwide will get us there. But as Rossi notes, “The biggest predictor of the success in HSCT is the number of cells transplanted. The more, the better chance that the graft will take.
“There’s a growing number of molecular experiments out there aimed at understanding the fundamental biology of HSCs,” he adds, “and out of that knowledge will come the information we need to have a huge clinical impact.”