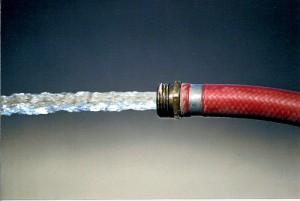
Blood should flow through an artery like water through a hose. The stress of a blockage can encourage clots to form, potentially resulting in a heart attack or stroke. Donald Ingber thinks the same forces could be used to help dissolve clots. (Beth Kingery/Flickr)
Grab a garden hose. Put your thumb over the end, but not all the way, and turn the water on. What happens? The water coming out of the hose gets squeezed as it tries to push past your thumb, putting a lot of force on the molecules in the water and making a big spray.
Now do the same thing with an artery: Partially block it with a clot and let blood flow through it. In this case, the force you’ve created in the artery could be lethal—creating fertile ground for blood clots that could lead to a stroke or heart attack.
But what if that combination of force and pressure could be used to stop something like a stroke instead? What if it could release a clot-dissolving drug on the spot? Donald Ingber, MD, PhD, a member of Boston Children’s Hospital’s Vascular Biology Program, had wondered that for many years. To find out, Ingber, who also directs the Wyss Institute for Biologically Inspired Engineering at Harvard, had his team start with a simple question: How do clots form?
Blood clots are basically tangles of proteins and cell fragments called platelets. When blood tries to squeeze past a fatty plaque (like one found in the arteries of people with cardiovascular disease), the force of the resulting shear stress activates platelets. Factors turn on that make the platelets sticky, causing them to clump together and stick to the plaque. This closes off the vessel even more, starving the tissues of oxygen and creating the pain or discomfort that we call a heart attack or a stroke.
“The shear stress in a blocked blood vessel can be 10 to 100 times greater than in a normal one,” Ingber notes.
Doctors can treat strokes or heart attacks with clot-busting drugs like tPA, either by injection or by finding the clot, threading a catheter to it and releasing a large dose right on top of it. Such drugs have to be delivered under careful watch in the hospital, because the doses required to degrade the clots can sometimes cause bleeding elsewhere in the body.
“There are great clot busting drugs out there,” says Ingber, “but the earlier you can give them, the better. We wanted to create something that an EMT could give on the scene at low dose which could target a clot and start dissolving it right away, but wouldn’t affect the rest of the body.”
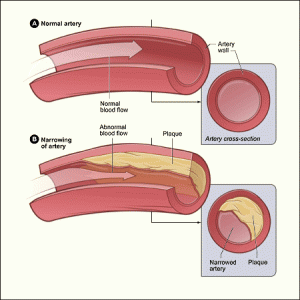
Cholesterol plaques create local spikes in shear stress in arteries that encourage clots to form. (NHLBI)
But how do you target a clot? Ingber’s solution: Do it the way a platelet does, by reacting to high shear stress.
His team coated a ball of nanoparticles the size of a platelet with tPA, structuring the ball such that it would hold together under normal blood flow and but break apart when exposed to high shear stress, releasing the drug only at the site of a clot or blockage.
“Imagine a ball of wet sand,” Ingber explains. “Just sitting in your hand, it holds together. But if you drag your hand over it, it breaks apart. That’s essentially what these nanoparticles do.”
And they do it quite well. When Ingber’s team tested the coated drug particles in mouse models, the nanoparticles broke up and delivered the tPA only where they encountered arterial clots in the lung. While these clots were fatal in untreated animals, 80 percent of those treated with the shear-activated particles survived, at 1/100th the normal clinical dose of tPA.
“You could see clots dissolve away in real time,” says Ingber. “We could even inject the particles a half hour after inducing clots with good results.”
Ingber notes that while his team is refining the technology, they’re looking to license it to a corporate partner or spin off a company to help bring it to patients. Either way, they aim to turn it into a tool that could be used to treat heart attacks, stroke and pulmonary embolisms without the side effects that limit tPA’s use.
“We also think the basic concept could work in diseases like sickle cell anemia, where red blood cells clump in small blood vessels, or for shear-triggered imaging agents for all kinds of vascular obstructions,” Ingber says.