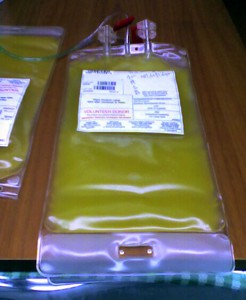
Researchers and doctors dream of being able to artificially produce platelets (in the blood bag above) at clinically useful scales. A device that mimics the environments in which platelets mature could help them get there. (Toytoy/Wikimedia Commons)
The platelet – a crucial cog in our blood’s clotting machinery – is in high demand. Trauma, chemotherapy, and surgery patients often need platelet transfusions to keep their blood working properly. So too do people with genetic disorders like Wiskott-Aldrich syndrome that prevent them from producing enough platelets on their own and cause thrombocytopenia.
However, platelets are in short supply compared to other blood products, in part due to their short shelf life.
“Platelets only last in the body for about 10 days at a time,” explains Jonathan Thon, a fellow in the laboratory of Joe Italiano, a member of Children’s Vascular Biology Program. “In a blood bank, red blood cells can be stored in a refrigerator for 42 days, and plasma can be frozen for years. But platelets need to be stored at room temperature, and only for a short time for fear of bacterial contamination.” Which means that few platelets are available for those who need them – a situation that screams for a means of artificial platelet production.
To make platelets, though, you need to understand, in minute detail, how they and their parent cells, called megakaryocytes, behave in the body. And to do that, you need to recreate, as much as possible, their normal living environment – or, in the case of a platelet, environments – and the stimuli they experience.
“Megakaryocytes start out in the bone marrow, a relatively solid and static environment with its own set of biochemical signals,” Thon says. “But as the cells mature, they gradually migrate to the bloodstream, which is liquid and dynamic, with fluids flowing at relatively high speeds. It’s a real shift, especially in terms of the shearing forces the cells experience when they get to the vasculature.
“With static cell culture, we’re not reproducing the full set of environments and forces well,” he continues, “which is probably why we’ve not been able to produce high numbers of clinical-quality platelets in the laboratory.”
But how to do so while maintaining the level of control needed to change one or two parameters at a time experimentally? Here we get into the realm of simulators that mimic the cellular environment, like 3D scaffolds and the lung-on-a-chip – complex systems for studying…well, complex systems.
For the last few years, Thon and his colleagues have been working on just such a system for solving the platelet production problem: A microfluidic device that could reproduce the platelet’s developmental milieu in a way that serves both researchers wanting to understand platelet development and the patients in need of lifesaving platelet transfusions.
The device, still in the proof-of-principle stage, features multiple chambers that can be coated with biochemical factors characteristic of different environments. It also incorporates microfluidic channels that allow Thon to expose single maturing megakaryocytes to the kinds of physical forces the cells experience at the interface between the solid bone marrow and liquid, flowing blood.
Thon has high hopes that the combination of flow and biochemical factors will speed up platelet production in the laboratory while revealing more about the biological mechanisms underlying platelet production.
“We also think that because we’re able to replicate gradients within the bone marrow, we might have a good system for studying fundamental features of hematopoiesis in general, not just platelet development,” Thon says. “With luck, it also may be possible to scale the system up to a bioreactor and produce large numbers of platelets for transfusion.”